Energy storage systems help us to realize the true benefits of renewables and advanced grid technologies. They mitigate intermittencies and unlock the potential of solar and wind power by storing clean energy as it is created, so that it can be used when it is actually needed. They help to support optimal operation of an increasingly complex grid, faced with increasingly more challenging peaks and dynamics associated with millions of distributed energy resources. Energy storage systems add resiliency to an electrical grid under increasing stresses from natural events and emerging threats like cyberattacks. They support responsible retirement and displacement of outdated energy assets as we build a more intelligent, modernized electrical infrastructure. And, they empower energy consumers to manage their energy use based on their individual priorities and values.
These systems can range from grid-scale systems that may be on the building level, to commercial systems that might be containerized to support a large building or microgrid, to residential-level systems for use in consumer applications. They might be owned and operated by electric utilities, governments, private parties, or consumers. Each of these solutions play a part in the overall expansion of the energy storage footprint as we move forward.
The mechanism used for energy storage can vary widely. They can employ mechanical means such as flywheels, thermal systems using compressed gases, potential energy systems such as pumped hydro, or systems using supercapacitors. However, the primary method used for private ESSs today is electrochemical storage in the form of battery systems, referred to as BESS. The battery technology can also vary, including flow batteries and more traditional lead-acid technologies. However, among these options, lithium-ion batteries are the chemistry of choice based on their energy density and performance.
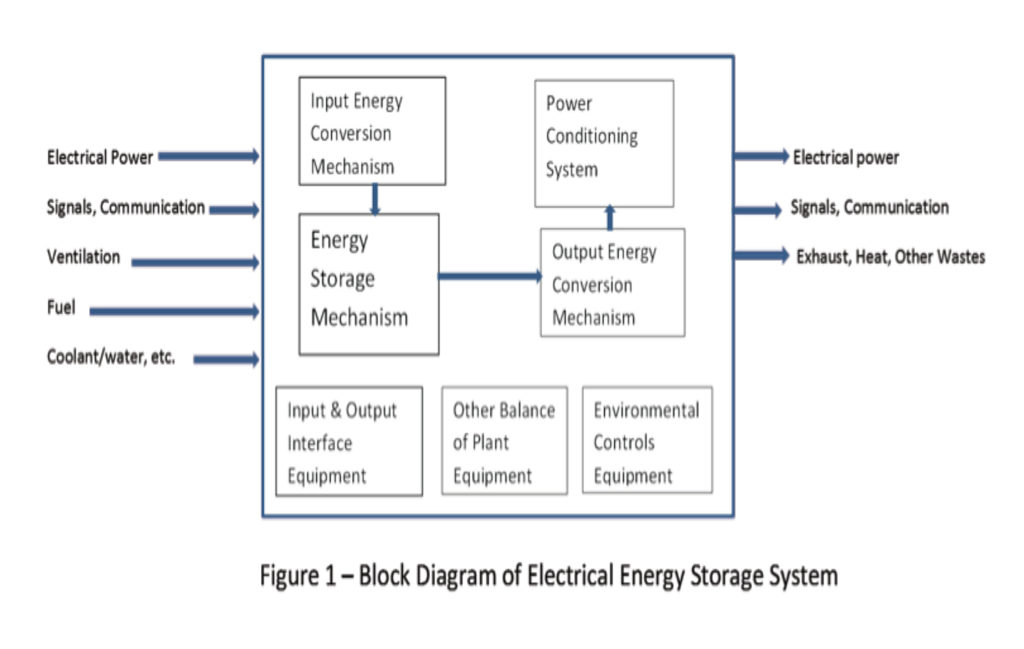
ESS safety
For any new technology to be sustainably deployed, safety must be foundational. To support the community in addressing this important aspect of ESS growth, several years ago, Underwriters Laboratories (UL) published UL 9540 Standard for Energy Storage Systems and Equipment, the world’s first Standard to address safety of ESSs. Now, the binational Standard for both the United States and Canada, UL 9540, addresses energy storage systems that are intended to receive electrical energy and then to store the energy in some form to provide electrical energy to loads or power conversion equipment when needed. The ESSs covered by this Standard include those intended to be used in a standalone mode (e.g., islanded), including “self-supply” systems to provide electrical energy; those used in parallel with an electrical power system or electrical utility grid such as “grid-supply” systems; or applications that perform multiple operational modes. UL 9540 also has been adapted in locations around the world, including India, and is supporting development of the international requirements for these products.
Safety of an ESS must consider a number of critical elements of the system’s operation. As shown in Figure 1, these installations are complex systems with numerous integrated subsystems. They may include equipment for charging, discharging, control, protection, power conversion, communication, controlling the system environment, air, fire detection and suppression system, fuel, or other fluid movement and containment, and other functions.
Addressing safety of an ESS necessitates consideration of a number of important factors. The method for importing power must be suitably coordinated. The means for storing energy must be thoroughly assessed for safety under both normal and abnormal conditions, which is especially important for technologies such as lithium-ion batteries. The power output must be appropriately delivered and controlled. This is particularly important for grid-connected systems, to ensure that the grid connection will not expose the system to vulnerabilities, the grid interconnection is suitable for the electric utility, and that the ESS will support the required advanced grid support features being depended upon increasingly as the grid evolves. Environmental factors often need to be addressed, especially when the systems are for outdoor use. Finally, human interfaces need to be addressed based on the unique aspects of the system design.
Of special concern for a BESS is the technological solution used to keep the batteries safe, known as the battery management system (BMS). It is essential for lithium-ion batteries, in particular, to have a carefully designed BMS that will keep the batteries in safe operating conditions throughout the various modes of normal and foreseeable abnormal operation. For example, lithium batteries that are not properly charged and discharged (or not kept in balanced charge) can present significant safety concerns. If the BMS does not effectively accomplish this type of limitation, lithium-ion batteries can go into thermal runaway—a dangerous failure mode where the heat within the battery increases in accelerating fashion. Thermal runaway often ends in fire or explosion of the lithium-ion cell, which can then induce thermal runaway in the surrounding cells. This type of “avalanche” effect can cause a single fault to cascade to a catastrophic event.
Other battery chemistries can have different, but equally important, failure modes that must be understood and mitigated. Compliance with the applicable safety requirements for the battery, such as the binational U.S. and Canadian Standard for Safety for Batteries for Use in Light Electric Rail (LER) Applications and Stationary Applications, UL 1973, is an essential step in understanding the behavior of the battery and supporting safe integration into an ESS.
For safety of an overall BESS, both safety of the batteries themselves and their compatibility with the BMS are critical. For this reason, it is essential, from a safety perspective, to have the BMS properly designed and validated through rigorous testing and review with the batteries that will be used. Battery management systems typically use a combination of hardware, firmware, and software to holistically accomplish their protective features. A thorough failure mode and effects analysis (often referred to as an “FMEA”) and a complete review of this functional safety system in addressing the FMEA are both needed to systematically verify the safety of the BESS. Only a BESS that has an integral, validated BMS that has been assessed with the specific batteries in use should be employed in the field, or significant hazards might emerge in the installation based on the inability of the system to keep the batteries in safe operating modes.
Energy storage system equipment may be constructed either as one complete system or as matched assemblies that, when connected, comprise the system. While an increasing number of ESSs are certified to UL 9540, for larger, customized systems, the most effective path to demonstrating compliance with UL 9540 and other applicable safety requirements is often through field evaluations.
Code developments
To support the proper integration of ESSs into the infrastructure, code development continues to evolve. These codes are leveraging the benefits of compliance with the safety requirements of UL 9540 on the system level and focusing on the safety of integrating the ESS into the electrical and physical ecosystems where they will be installed.
Several changes were implemented in the 2017 NEC related to energy storage systems. Section 480.3 was added to specify new requirements for listing of storage batteries and BMSs for chemistries other than lead-acid batteries. Also, a new Article 706 was added to the 2017 NEC to cover electrical safety of ESS installations. These requirements address important integration requirements such as properly sizing and protecting circuit conductors for more various modes of operation, spaces about ESS electrical equipment, properly identifying the presence of multiple sources of electrical power, and disconnection means. Article 706 continues to evolve as we enter the final stages of the 2020 NEC development process.
The 2018 edition of NFPA 1 was updated to include references to UL 1973 and UL 9540 and to require listing of advanced battery technologies and systems to UL 1973 and UL 9540. NFPA 855, Standard for Energy Storage System Installation, is also being developed to address overall installation aspects of ESSs. NFPA 855 applies to the design, construction, installation, and commissioning of ESSs. This development work is being pursued aggressively and is expected to be completed in 2019 for a 2020 first edition.
The 2018 International Fire Code included significant revisions to Section 1206 to address energy storage systems, specifically battery systems. To address concerns for fire spread, these codes have limitations on installation of BESSs for indoor installations in non-dedicated use buildings and outdoor installations near exposures such as non-dedicated use buildings. Based on the types of systems used and the level of compliance with safety standards that has been established, there are differentiated requirements for limitations on the size of the BESS, separation distances to limit propagation of fire events to or from a BESS to another BESS or to the structure, limitations on the floor level where the systems will be installed, and completion of a hazard mitigation analysis. Again, significant work is presently underway to evolve these approaches for the next edition of the International Fire Code.
BESS fire safety
Because of the possibility of a BESS that is engaged in a fire event cascading to a larger scale fire, there has been significant work done to address the underlying safety science associated with this scenario. UL has conducted fire safety research that addresses large scale fire testing methodologies for BESSs. This work has been captured in the requirements of Test Method for Evaluating Thermal Runaway Fire Propagation in Battery Energy Storage Systems, UL 9540A.
The test method of UL 9540A evaluates the fire characteristics of a BESS that undergoes thermal runaway. It provides methods for a holistic assessment of fire performance of a BESS through the definition of multiple levels of testing. Testing can be done at the cell level, where the consequences of a single cell failure is assessed with respect to the risks of cascading to a larger fire event on the system level. Module-level tests address the same concern involving failure of a larger module comprising multiple cells. Unit-level testing repeats cell failure in one of the modules in an initiating BESS unit under test and assesses the interaction of that surround with target BESS units at defined distances. Installation-level tests consist of repeating the unit-level test within a test room with defined sprinkler systems to gauge the efficacy of suppression.
Testing at each of these levels provides critical safety information that supports informed, appropriate decisions about the safety of the BESS and how to best integrate these systems safely into a given installation. Results will identify macro-level performance, such as if fire propagation or explosions occur. Results also provide a collection of important data such as content of gases emitted, heat release rates, and heat flux and temperatures at target surfaces. This data is used to determine the fire and explosion protection required for an installation of a BESS in accordance with applicable codes and approval processes.
The future of energy storage
As we look to the future, we see that this very dynamic sector will continue to evolve in even more interesting ways. Certainly, battery chemistries will continue to evolve in the future, as we look for optimal performance of battery systems. The scientific and safety engineering communities continue to work to support that process unfolding with safety as a key concept. Chemistry agnostic safety standards such as UL 1973 and UL 9540 will support appropriate customized assessments of the unique hazards associated with these new chemistries as they actually come to market in scalable form.
The continued convergence of the automotive fleet and the electrical infrastructure continues to play out in very interesting ways that will impact energy storage as we go forward. “V2X” technology, which supports power export from an electrified vehicle (battery electric vehicle or fuel cell vehicle) to a load (V2L), to a home (V2H), or to the grid (V2G), is being used around the world and is likely to find specific niches in North America, especially related to dormant electrified fleets that comprise a large deployable bank of energy storage assets. We are also seeing growth in uses for repurposed electric vehicle (EV) batteries.
As EV batteries age, they face limitations in charging capacity that impair continued use in motive applications and trigger the end of their useful life within an EV. However, these batteries can still retain significant capacity, on the order of 80 percent of their original characteristics, and can be useful if repurposed into the energy storage infrastructure. Making sure these cells, modules, and battery packs are safe, suitable for their continued use, and suitable for repurposing into an infrastructure application all need to be addressed.
The requirements of the newly published binational U.S. and Canadian national safety standard, Evaluation for Repurposing Batteries, UL 1974, will support safe integrations of these repurposed EV batteries into their new second lives. Similarly, the H2@ Scale Initiative being advanced by the U.S. Department of Energy—along with support and help from the industry—demonstrates how hydrogen can be used as a critical, dispatchable medium to support electricity generation, transportation, and energy storage in an innovative new ecosystem.
Energy storage is already being rapidly integrated into our world, and we will all see an increasingly visible impact in our communities as we move forward. Energy storage technologies will enable a more intelligent, cleaner, more resilient energy future in many varied ways. Significant work that has been done to research safety issues, develop safety standards, and advance Code requirements for safe integration of ESS all provide an important foundation for safe deployment of these systems. By leveraging the benefits of this underlying scientific and engineering work, the inspection community will play a critical role in making sure we have safe energy storage everywhere.
For more information on UL Standards, visit www.ul.com/batteries.
Find Us on Socials