The topic of grounding and bonding as it relates to the National Electrical Code (NEC) and proper installations still seems to be a mystery to many electricians, engineers and inspectors. In the author’s travels around the U. S. and Canada, this seems especially true as it relates to services, separately derived systems, and when another building or structure is served by a feeder or branch circuit. The NEC Task Group on Usability started a process that caused Article 250 to be totally reorganized for the 1999 cycle to help users find the requirements easier. During the 2002, 2005 and 2008 cycles, Code-Making Panel 5 has continued on that approach to clarify text and continue to improve the requirements for better understanding. As the next wave of technology, including photovoltaic systems, wind generators, electric vehicles, and the smart grid, is now rapidly evolving, it is even more important to all the electrical industry to have a firm grasp on the fundamentals, including the foundation principles of grounding and bonding. This article will focus on separately derived systems and the requirements found primarily in Section 250.30 ofNEC-2008. In addition, some previews of proposals under consideration will be mentioned as CMP-5 is processing the 2011 code cycle.
What is a separately derived system? The immediate answer is found in the Article 100, Definitions. One of the changes that occurred during the 2005 NEC cycle was that some of the definitions in Article 100 were reassigned from Code-Making Panel 1 to specific code panels, where the term being defined was more appropriately under their purview. Many definitions related to grounding and bonding were assigned to CMP-5 and this now includes the definition of separately derived system. The definition as published in NEC-2008 is:
Separately Derived System. A premises wiring system whose power is derived from a source of electric energy or equipment other than a service. Such systems have no direct electrical connection, including a solidly connected grounded circuit conductor, to supply conductors originating in another system.
Photo 1. Transformer separately derived system
To understand this definition, we need to look at the two interrelated parts. The first sentence tells us that we have a premises wiring system that is from a source of energyother than a service. This source can be many things as will be discussed later. For some of the “old timers” we need to review and remember that aservicenow comes only from a utility. This is one of those changes that has taken some time for many experienced installers to fully adopt and to change how they reference the source of electrical power to a premises wiring system. The Article 100 definition provides an explanation of whatpremises wiringmeans as the “Interior and exterior wiring, including power, lighting, control, and signal circuit wiring together with all their associated hardware, fittings, and wiring devices, both permanently and temporarily installed.”And further it includes
(a) wiring from the service point or power source to the outlets or
(b) wiring from and including the power source to the outlets where there is no service point.”
Since the definition of a separately derived system tells us that the source is from something other than a service, the part “(b)” above applies for that aspect of the separately derived system. It is a common case for a premises wiring system to be installed and there not be a service at all. Think of a relocatable rock crusher operation supplied only from a generator on a trailer.
Photo 2. Generator separately derived system
The second sentence of the separately derived system definition goes on to state that there is no interconnection of conductors that carry current under normal conditions between this “other source of energy” and the “service” or possibly another source of energy. The statement goes on to specifically say that the grounded circuit conductors (neutrals), if they exist in either system, are not solidly connected together. In other words where they exist, they are switched between sources of energy just like the ungrounded conductors are switched between sources. One thing to note, the interconnection of equipment grounding conductors, the grounding electrode system, or bonding conductors from one source to another does not mean there is not a separately derived system.
Some examples of separately derived systems would include:
- Transformers, shown in photo 1, where the supply side is isolated from the derived side except by magnetic coupling
- Generators (motor, wind, or engine driven), one example shown in photo 2 and figure 1, where it is either a totally stand-alone system or is an alternate source of power and the grounded conductor (neutral) is not solidly connected in the transfer switch or transfer equipment
- Battery/inverter systems where the output is not interconnected
- Photovoltaic systems where there is no interconnection to the grid or another energy source (off grid system)
Once it has been determined that we are dealing with a separately derived system, then we need to look at the requirements for grounding and bonding. For the purposes of explaining these requirements, we will use a 75-kVA 480-volt delta to 208/120-volt wye transformer as our ongoing example. The core requirements for how to complete grounding and bonding of separately derived systems are found in 250.30 of NEC-2008. Section 250.30 is located in Part II of Article 250 under “System Grounding.” Part II is where the system grounding requirements would be found for AC systems, including services (250.24), separately derived systems (250.30), buildings or structures served by feeders or branch circuits (250.32), portable and vehicle-mounted generators (250.34), permanently installed generators (250.35), and high-impedance grounded systems (250.36).
Figure 1. Generator as separately derived system
Before getting into the details of how to provide the grounding and bonding, the question “to ground or not to ground the system?” has to be answered. This determination of if the separately derived system is required to be grounded, may be optionally grounded, or is prohibited from having the system grounded is found in NEC-2008 Sections 250.20, 250.21, and 250.22. Section 250.20 covers AC systems that are required to be grounded and 250.20(D) specifically addresses separately derived systems. Section 250.20(D) establishes that systems identified in 250.20(A) or (B) are to be grounded in accordance with 250.30(A). Our example system would fall under 250.20(B)(1) and must be grounded; therefore, we now need to follow the requirements in 250.30(A). In the event the separately derived system was covered in 250.21, a decision has to be made on if it is desired to ground the system or to leave it ungrounded. If the decision is made to ground it, then again we move to 250.30(A) for the requirements; but if it is decided to leave it ungrounded, then the frames of the derived system would be connected to ground to meet 250.4(B) and ground detectors as required by 250.21(B) would be required to be installed.
Having completed the above and moving to the requirements for grounding and bonding, there are five distinct steps to be completed for grounding a separately derived system. Section 250.30(A) is generally structured to complete these steps in a logical manner considering the interrelationship of some aspects in each step. The rest of this article will focus on each of these steps.
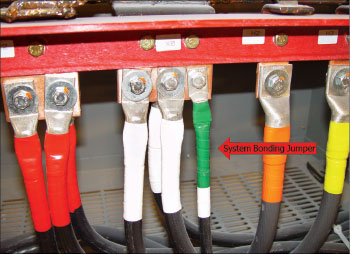
Step 1. 250.30(A)(1), System Bonding Jumper
Figure 2. System bonding jumper at source of separately derived system
There are some exceptions that permit alternatives under given conditions. Section 250.30(A)(1) Exception 1 permits a single system bonding jumper connection where the system is dual fed or double ended. As indicated above regarding a special case allowing two connections to ground on the neutral, 250.30(A)(1) Exception 2 does permit the use of two system bonding jumpers, one at the source and the other at the first disconnect enclosure where to do so does not create a parallel path for normal neutral current to flow on the grounding conductors. An example of this could be an outdoor padmount transformer serving a building switchboard, using non-metallic raceway(s) as the wiring method and not having equipment bonding conductors installed.
The system bonding jumper, like the main bonding jumper in a service, is required to be of a material, size and installation per 250.28(A) through (D). It is to be copper or other corrosion-resistant material, and wire or busbar types may be bare, insulated or covered. It should be noted here that there is no identification requirement, such as a color, for wire or busbar type system bonding jumpers in the NEC. Section 250.119 would permit the use of the color green, and that is a typical marking, but field-installed identification is not required. Section 250.28(B) and the UL product standards for listed equipment, specify that where the main or system bonding jumper is a screw, it must be colored green. In dead-front switchboards, the product standards go on to require where a wire or busbar type main bonding jumper is installed at the factory, it must be marked with an identification label.
Figure 3. System bonding jumper at the first disconnection means
The system bonding jumper is to be unspliced and generally both terminations are within the enclosure containing the source or first disconnect depending on the installation location chosen. Terminations are to follow the requirements in 250.8 using connectors listed under UL categories Wire Connectors and Soldering Lugs (ZMVV) located on page 434 in the 2009 UL White Book or Grounding and Bonding Equipment (KDER) located on page 194 in the 2009 UL White Book.
The system bonding jumper size is based on the derived phase conductors installed from the source to the first disconnecting means. To size the system bonding jumper, find the size of the largest ungrounded conductor and use Table 260.66 to find the appropriate size, copper or aluminum, conductor for the system bonding jumper. Where the largest derived conductors are larger than 1100 kcmil copper or 1750 kcmil aluminum, then the system bonding jumper shall be a minimum of 12½ % of the cross sectional area of the largest ungrounded conductor. For our example, the ungrounded phase conductors are 3/0 copper, so the system bonding jumper would be 4 AWG copper or 2 AWG aluminum.
Photo 4. Transformer core ground not to be removed
A couple of final notes about the system bonding jumper. If it is decided that the grounding and bonding is to be completed at the first disconnecting means, a factory supplied system bonding jumper in the transformer can be removed or on most generators can be relocated without impacting the UL listing. Some generator models have a permanent bonding jumper so follow the manufacturer’s instructions when relocating and making any changes to a generator bonding jumper. It is also important that the transformer core bond, photo 4, not be removed. If you remove the system bonding jumper strap or wire, be sure it is the connection between the neutral (commonly identified as Xo) and the enclosure.
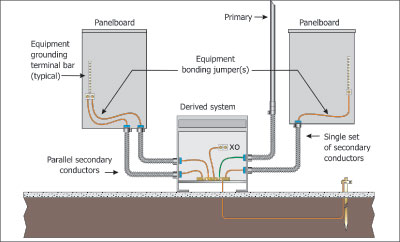
Step 2. 250.30(A)(2), Equipment Bonding Jumper
The function of this conductor is to carry ground-fault current between the source and the enclosure with the first disconnecting means to provide the effective ground-fault current path and meet the requirements of 250.4(A)(5). When the system bonding jumper is installed at the source, this bonding jumper provides the low impedance path from the equipment grounding conductors in the disconnect panel to the system bonding jumper at the source and then to the Xo terminal to complete the circuit.
When the system bonding jumper is installed at the first disconnect panel, the ground-fault current from a load will travel on the equipment grounding conductors to the panel and onto the system bonding jumper and then to the system grounded (neutral) conductor. Section 250.30(A)(8) provides additional requirements for installation and sizing of the neutral conductor for this situation. It would seem the equipment bonding jumper is not needed since it is not providing the normal fault current path as the situation above. The case where it is needed happens when the source, secondary windings of the transformer in our example, experiences a ground fault. The fault current needs a low impedance path back to the first disconnect enclosure to then flow on the system bonding jumper and back to the source on the neutral conductor. Both these current paths, shown in figure 5, are to facilitate the operation of the appropriate overcurrent device.
As a preview to some of the 2011 NEC activities, it was discovered during vigorous discussions that the use of the term equipment bonding jumper in this application was incorrect. By definition theequipment bonding jumper continues the path to connect two or more equipment grounding conductors. Since our case is on the supply side of the overcurrent device, we don’t have equipment grounding conductors so we obviously cannot be completing or extending their installation. A new term has been proposed for the case of bonding conductors on the supply side of overcurrent protection for both services and separately derived systems. The new term is supply side bonding jumper or SSBJ. All the requirements for installation and size remain the same, but the new term should help eliminate any confusion about using equipment bonding jumper for more purposes than the definition really allows.
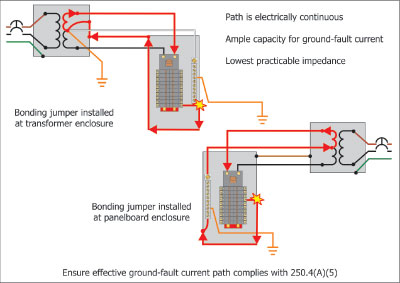
Step 3. 250.30(A)(3), 250.30(A)(4) and 250.30(A)(5), Grounding Electrode Conductor
There are two main options for installing the GEC provided, option one is an individual GEC [250.30(A)(3)] from each separately derived system to the grounding electrode or grounding electrode system, or option two is a common grounding electrode conductor [250.30(A)(4)] where taps are connected to the individual separately derived systems. In both cases, the connection point for the GEC at the source or the first disconnecting means enclosure must be the same location as where the system bonding jumper was installed in step 1 above. Both options also require the grounding electrode conductor to be installed per 250.30(A)(5) which requires that all the requirements in 250.64(A), (B), (C), and (E) be complied with for this installation.
Figure 6. Listed assembly with single grounding electrode conductors
As permitted for the system bonding jumper, both these options provide an exception for dual source or double-ended equipment to have one grounding electrode conductor for the assembly installed at the tie point. Also, both methods, as provided in 250.30(A)(3) Exception 2 and 250.30(A)(4) Exception, permit a single grounding electrode conductor to serve both for an equipment and a built-in separately derived system as shown in figure 6. The listed equipment assembly must be identified as “Suitable for Use as Service Equipment” and the grounding electrode conductor must be sized to the larger of the requirements for the equipment, such as at the service, or for the separately derived system derived phase conductors.
Option 1 uses the requirements in 250.30(A)(3). If an individual GEC is to be installed, it shall be sized per Table 250.66 based on the size of the largest ungrounded conductors of the derived system. It would be connected at the source, our example transformer, or enclosure with the first disconnecting means to the grounded (neutral) conductor and at the other end, the GEC is connected to a grounding electrode or the grounding electrode system. The choices for grounding electrodes will be discussed in step 4.
Photo 4. Transformer core ground not to be removed
Exception 1 to 250.30(A)(3) permits the grounding electrode conductor connection at the source, our example transformer, to be to the equipment grounding terminal bar or bus where the system bonding jumper from the equipment grounding terminal bar or bus to the neutral is a wire or busbar. An example is shown in photo 4.
Option 2 uses the requirements in 250.30(A)(4). A relatively newer provision in theNECallows a practice that building design engineers have used for many years. Large buildings, such as high-rise structures typically have multiple separately derived systems. For example, a high rise may have a 480-volt bus riser that has disconnects on every floor to serve a step-down transformer, like our example, that supplies 208/120-volt utilization power to receptacle and equipment on that floor. To provide an effective means for grounding all these systems, what is now called acommon grounding electrode conductoris installed, typically, along with the electrical riser to each floor’s electrical room. The common grounding electrode conductor is connected to the building’s grounding electrode system in a suitable location. The individual separately derived systems are then connected to this common grounding electrode conductor by taps as shown in figure 7. This provision for multiple separately derived systems is optional for use and if it is used, then the provisions of 250.30(A)(4) apply; otherwise, use 250.30(A)(3) and a separate grounding electrode conductor for each separately derived system.
Figure 7. Common grounding electrode and tap connections
The size of the common grounding electrode conductor is set as a minimum 3/0 copper or 250 kcmil aluminum. The common grounding electrode conductor, in accordance with 250.64(C) must be unspliced or if it is spliced, the splice be by exothermic welding or by the use of irreversible crimp type connectors listed as Grounding and Bonding Equipment, UL category code (KDER), located on page 194 in the 2009 UL White Book. The tap conductors can be connected to the common grounding electrode conductor with any of the connectors identified in 250.8, UL categories ZMVV or KDER, or to connectors on a copper or aluminum busbar with minimum dimensions of ¼ by 2 inches and sufficiently long for the connections to be completed. The tap conductors are sized for each separately derived system based on the largest derived phase conductor(s) for that system. For our example transformer, the tap conductors would be 4 AWG copper or 2 AWG aluminum and we will connect to a copper bus with standard Listed (ZMVV) mechanical pressure connectors where the common grounding electrode conductor is connected by a lay-in type terminal listed (KDER) connectors for grounding and bonding.
Step 4. 250.30(A)(7), Grounding Electrode
With one end of the grounding electrode conductor installed at the separately derived system and routed and protected as required in 250.64, it must now be connected to a grounding electrode or some suitable point on the grounding electrode system. Section 250.30(A)(7) specifies the grounding electrodes to be used and also indicates a preference or priority. The grounding electrode selected “shall be as near as practicable to and preferably in the same area as the grounding electrode conductor connection to the system.” The preferred grounding electrodes are:
1. a metallic water piping system meeting the requirements of 250.52(A)(1) including the exception, or
2. interconnected structural metal frame making up the structure of the building as specified in 250.52(A)(2).
In addition to setting these two electrodes up, as preferred, 250.30(A)(7) sets a priority by requiring that the closest one of the above be used as the grounding electrode.
Another preview to proposed changes in the 2011NECis the recognition of the structural metal frame of a building as another type of “common grounding electrode conductor” and to differentiate structural metal in the earth, truly an electrode, from the metal in the building or structure that is acting as a conductor.
Where neither of the above electrodes is available, then the option to use any other electrode identified in 250.52 is allowed. The connection of the grounding electrode conductor to the grounding electrode is to be completed with listed connectors and as specified in 250.68 and 250.70.
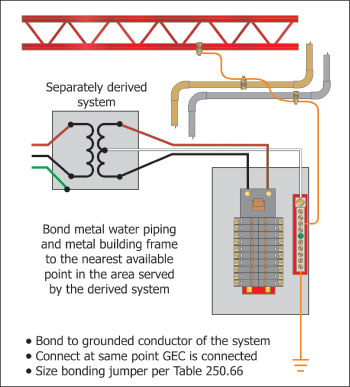
Step 5. 250.30(A)(6), Bonding
If the metallic water piping system was used for the grounding electrode, then no additional bonding connection is required. The installed grounding electrode conductor to the metallic water piping serves both functions to provide the ground reference and also to act as the bonding conductor. Similarly, if the structural metal was used as the grounding electrode, then no additional bonding connection is required. The installed grounding electrode conductor to the structural metal serves both functions to provide the ground reference and also to act as the bonding conductor. In the case where another electrode or the common grounding electrode conductor was used, because the local metallic water or exposed structural metal was not suitable as a grounding electrode, then both the metallic water piping and the exposed structural metal would need to be bonded to the separately derived system.
This can be accomplished in several ways. Since bonding conductors are not required to be continuous, i.e., they can be daisy-chained, then the structural steel can be bonded to the water piping and the water piping in turn bonded to the separately derived system as shown in figure 8. Another option exists where a number of separately derived systems may exist in an area. For example, if the common grounding electrode busbar scheme is employed for two or more separately derived systems, it would be acceptable for the metallic water piping and the exposed structural metal to be bonded to the common grounding electrode conductor busbar to effectively bond these separately derived systems. The only provision would be that the bonding conductors used would have to be sized for the largest of the derived phase conductors from the separately derived systems in the area served.
250.30(A)(8) – Grounded Conductor (Neutral)
This last provision in 250.30(A) covers the case where the system bonding jumper is located at the first disconnecting means. For this installation, as discussed above in step 2 regarding the ground-fault return path, the grounded conductor (neutral) must carry the ground-fault current as well as any neutral current that might be present. Section 250.30(A)(8) provides that the size of the grounded conductor be sufficient to carry a fault current similar to the provisions in 250.24 for services. Where the system bonding jumper is installed at the first disconnecting means enclosure, the grounded system conductor from the separately derived system source to the first disconnect enclosure shall be sized for the larger of the expected neutral current per 220.61 or per Table 250.66 including the 12½ % rule. There are also provisions for when the grounded conductor is installed in parallel in separate raceways or when the separately derived system is impedance grounded.
For our transformer example, 220.61 calculations may determine a neutral size, but 250.30(A)(8) would require the absolute minimum size to be 4 AWG copper or 2 AWG aluminum.
In summary, there are many considerations when dealing with the grounding and bonding of separately derived systems. First, is that we need to consider the power source and determine if it is in fact a separately derived system. Once that is done, we can apply the steps as discussed above to provide for the system bonding jumper, the equipment bonding jumper, the grounding electrode conductor, the grounding electrode selection, and finally the bonding provisions as needed. Over the last fewCodecycles there have been significant improvements in organization and changes to text to clarify for users what the options are and what the requirements for each of those options entail. Installations may have unique conditions but theCoderequirements provide guidance for the designer, installer, and inspector to ensure the separately derived system is installed to be code-compliant.
Find Us on Socials